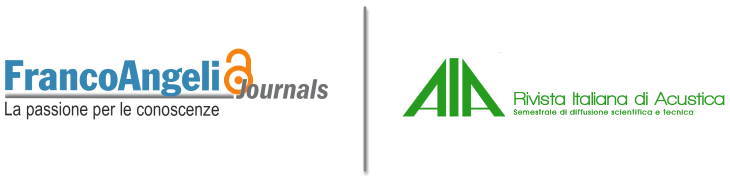
Human impact and the increasing energy demand urge the adoption of renewable technologies able to convert or recover unused energies, such as industrial or solar waste heat. In this context, thermoacoustics emerges as an effective sustainable solution, utilizing low-temperature sources with eco-friendly fluids, and is advantageous for its low operational and maintenance costs. The core of this technology is the stack, which is a porous material essential for the viscous and thermal interactions necessary for energy conversion. This research aims to optimize the efficiency of thermoacoustic engines and refrigerators by employing unconventional stacks such as Tetragonal Pin Arrays, Wire Mesh, and 3D Membrane Foams. Using a finite element numerical method, the transport parameters of the microstructures are characterized to develop predictive models of thermoacoustic behaviour, validated with new acoustic measurement techniques. Finally, a preliminary analysis reveals the potential of these innovative materials in enhancing the performance of thermoacoustic devices.